Multiplication by Divisions
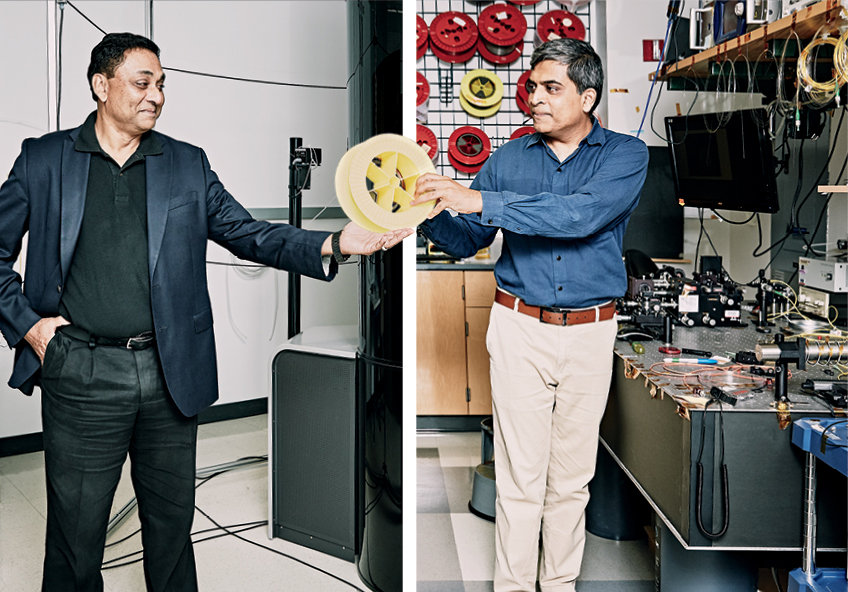
Materials, Systems Divisions Cross Traditional Barriers to Facilitate Research
Fuel cell use could be one of the best ways to mitigate climate change—fuel cells work like batteries, provide efficient power and don’t emit air pollutants. But there are multiple barriers in research and development before they will be available to a commercial market.
Professor Soumendra Basu (ME, MSE) has been working on fuel cells, but, along with everyone else, was stymied by one of those barriers: the material used on the cell’s cathode was unstable and subject to decomposition. For help, he reached out to Professor Karl Ludwig in the Physics Department.
“We have been using really state-of-the-art methods to look at just the surface of these materials to figure out how stable they are,” Basu explains. “And that’s where we collaborate with surface scientists from physics. We bring in the materials and device expertise here in engineering, and the surface scientists bring in the expertise to look at only a very, very thin surface to figure out what’s going on.”
“We really made some contributions in understanding the instability of the surface, and this collaborative effort showed how not only the composition of this material, but also how strained it is, plays a role in this surface instability.”
The connection between engineer and physicist was facilitated by their membership in the College of Engineering’s Materials Science & Engineering (MSE) Division, a research-focused unit that includes 87 faculty members from several BU schools.
Their ability to work together and even supervise the same graduate students comes from the MSE Division, without which, this work may not have been successful.
INNOVATING AT EVERY LEVEL
The MSE and 系统工程 (SE) Divisions are built on a unique concept.
“The idea was to create these divisions that would cut across departments and act as a glue among people that may be in different departments, but those people have a common technical language, and do work in the same application space or work that is driven by similar methodologies,” says Professor Ioannis Paschalidis (ECE, BME, SE).
Shortly after assuming the deanship, Kenneth R. Lutchen initiated a review of the academic units with an eye toward aligning the college’s strengths with emerging societal needs; among the results was the creation of the two interdisciplinary divisions that complement the traditional academic departments of Biomedical, Mechanical and Electrical & Computer Engineering.
Systems engineering and materials science & engineering are two extremely versatile disciplines, and can be applied to almost every field, systems dealing with the more abstract practice of processes and optimization, and materials with the physical world and what things are made of. Not only are both SE and MSE subject matters unique, but so is the concept of a division. Each faculty member who has an appointment or affiliation in one of the divisions also has a primary appointment in a traditional academic department, which can be within or outside of the College of Engineering. The two main division missions are to act as research units and to award master’s and doctoral degrees, which distinguishes them from discipline-specific departments that also award bachelor’s degrees (although undergraduates can minor in a division), and from research centers that do not award degrees.
“It was really a visionary thing for Engineering to do at the time,” Paschalidis offers. “I think it amplified the strength of the college because we are a particularly small college compared to the rest of the University, but also compared to other institutions. It allowed us to enhance interdisciplinary work, create bridges between departments, and reduce the height of the barriers between departments.”
Division heads Professor David Bishop (ECE, Physics, MSE, ME, BME) and Professor Christos Cassandras (ECE, SE) agree.
“When the two divisions were formed, they had to establish themselves in terms of research and build an identity of their own right,” Professor Michael Caramanis (ME, SE) explains. “It took some time, but by now they have managed to be quite successful.”
Success in this case means increasing the size of their faculty, funding and enrollment—all indications that the research is thriving—which over the past 11 years, both divisions have done. Appointed and affiliated faculty across both divisions now totals 118, up from 87 in 2008; funding in SE has increased from $29 million in 2008 to $47 million in 2017, and has skyrocketed in MSE from $22 million to $82 million in the same time period. Graduate enrollment is up, too, from 32 to 67 in SE, and from 20 to 72 in MSE from 2008 to 2017.
Perhaps the most illustrative sign of success has been the numerous research collaborations within the divisions. Sparked in different ways, these projects range from one researcher lending expertise to another, to large grants that support research projects spanning departments and colleges.
Both divisions have recently helped secure multimillion-dollar, collaborative grants that involve other colleges at BU as well as other universities. In 2018, Bishop spearheaded the effort that won the University the $20 million National Science Foundation (NSF) Engineering Research Center with the goal of creating personalized heart tissue for clinical use. And this year, Paschalidis led a group as the principal investigator (PI) that was awarded a $7.5 million grant from the Department of Defense for developing bio-inspired autonomous control systems.
Cassandras believes that all engineering research is headed down the kind of interdisciplinary path the divisions were created to pursue. “From a research standpoint, the divisions make complete sense because all of engineering has become interdisciplinary,” he points out. “That’s why you see all of these multi-PI projects with people from many different departments. Twenty years ago it would happen, but it was rare. Now, it’s the rule. If anything, we are going to see more divisions like SE and MSE because of how intertwined research has become.”
BUILDING BRIDGES
Professor Soumendra Basu (ME, MSE) remembers a chance encounter with Professor Siddharth Ramachandran (ECE, MSE) at a division seminar being the catalyst for a collaboration that grew from a discussion of an NSF grant.
“The materials science division had a speaker come in and talk about optical fibers, and our resident expert is Siddharth,” says Basu, MSE associate director. “I knew him well, but we never really talked science. So we attended the seminar and then we were just talking outside and he says, ‘Yeah, this is a big problem. There really are no good fibers for mid-infrared range. All the communication fibers are in the visible wavelength.’”
Basu asked him why that was, and the two delved into the problem and began to discuss possible solutions. But research on such novel ideas is all dependent on funding, and since large funding agencies like the NSF generally require at least some preliminary results that attest to the research’s promise, the college offers seed-money grants like the Dean’s Catalyst Awards to help get such projects off the ground.
After winning seed grants from the division and the college, Basu and Ramachandran began work, then applied for an NSF grant and were awarded more than $440,000 to continue the research.
“Nobody is more surprised than I was because I knew nothing about optical fibers, I just knew about processing,” Basu admits. “So this seminar and the division got two people with two totally different areas of expertise together.”
In the end, their project produced the most efficient fiber for the mid-infrared range.
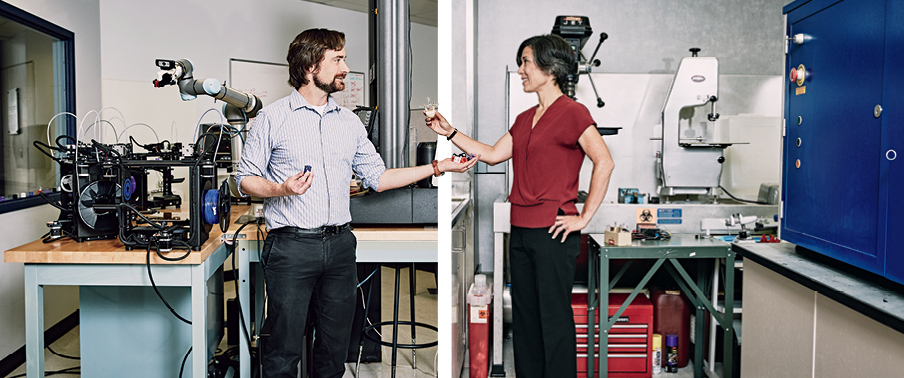
Even within departments, expertise can vary. Both Professor Elise Morgan (ME, MSE, BME) and Assistant Professor Keith Brown (ME, MSE, Physics) have primary appointments in Mechanical Engineering, the former in the mechanical behavior of biological materials and the latter in nanotechnology and soft materials.
Their research collaboration explores an autonomous method to engineer tough hierarchical materials, a project facilitated by the MSE division. Like Basu and Ramachandran’s collaboration, Morgan and Brown secured a Dean’s Catalyst Award.
Brown’s lab focuses on developing new methods, typically tools, to investigate properties of polymers and soft matter at the mesoscale—the level of matter in between micro- and macroscopic (around 0.1mm to 1mm). He is motivated by the lack of knowledge about the properties of materials at that scale, which is driven by the absence of the necessary tools.
“I was thinking about a 3D-printing system where you can create a structure, test it and then learn from it,” he says. “So if you’ve got an autonomous system you could give it a hypothesis, something like, ‘I want to know what kind of structure is going to have the best property.’ Then it will automatically test structures, and tell you the answer.”
“It became clear to me that one of the most interesting questions no defects. And defects are almost impossible to prevent during even substitutes in the future Vajda says that although this work has direct implications in the you can ask is, How do materials fail?” he continues. “Failure is really interesting because it’s very hard to simulate. It’s pretty clear how a material or structure is going to behave, but as you push it to the point where it starts to crumble, it becomes much more complicated. And so experiments are really necessary to test it.”
Brown knew that Morgan’s expertise was in bone biomechanics: “And, of course, one of the things we worry about with bones is them breaking, right? So we started talking about how we can learn about different failure mechanisms from an automated researcher.”
As of now, they have completed work on the autonomous system and are beginning to use it to test structures. The system will print a 3D structure, remove it from the printer, put it on a scale, and crush it. It will record every detail of the process, creating a vast database of structures and data around how they behave when they are crushed and potentially experience failure.
“The idea is if you have a lot of data coming in, you can use deep learning approaches to recognize structures that have done better with specific features and explore that region more,” Morgan says. “The vision is to do such a large volume in the course of a day, whereas it might have taken human researchers months to test that volume.”
And the property that Brown and Morgan first chose to explore is toughness, which is directly related to failure.
Toughness and strength are also related, but separate properties. Glass, she says, is actually stronger than steel–but only when it has no defects. And defects are almost impossible to prevent during even the most advanced manufacturing.
“If you have glass with a scratch on the surface, it’s going to fracture from a much smaller load than steel,” she explains. “Steel is much more defect tolerant, meaning it’s much tougher than glass.”
“Over many centuries of work in materials and in mechanical engineering more broadly, we have gotten good at designing, fabricating and manufacturing strong materials, but designing tough materials has definitely lagged behind,” she says. “But it turns out that nature’s pretty good at designing tough materials. Bone, for instance, is surprisingly tough, considering what it’s made of.”
The bone itself is made of mineral, which is pretty brittle—tough but not strong. It’s attached to collagen, which is stretchy and strong but not necessarily tough. Together, these two materials create a synergistic structure that boosts the toughness of the entire system. But the way those two components work together is complicated and not well understood.
“With advances in additive manufacturing, you make very complicated hierarchical component designs,” Morgan notes. “The rate-limiting step to find the best design is testing them and evaluating the results, but the advances in autonomous systems and robotics have enabled us to open up that rate-limiting step quite a bit.”
Morgan says that these new structures could be used for protection, such as pads inside of a helmet, and potentially synthetic bone substitutes in the future.
The entire system—including the robotic elements and the software—took about a year to build. They also pulled in another faculty member, Assistant Professor Emily Whiting (Computer Science), to help with the computational algorithm development. The three applied for and were awarded a Research Incubation Award from BU’s Rafik B. Hariri Institute for Computing and Computational Science and Engineering.
In order to have the system be autonomous and not just automated, it needed to run machine learning algorithms during the testing and evaluating phases. While the system runs, it learns about failure in 3D-printed parts, and can choose the next design to test based on past results.
CREATING SPACE
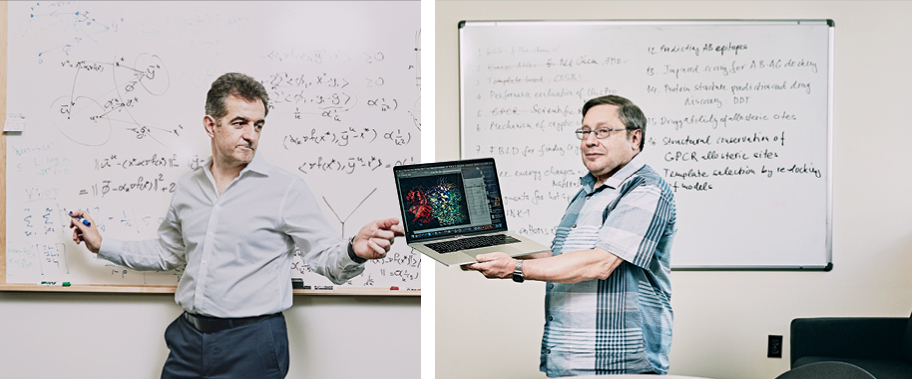
In addition to creating new collaborations, the divisions have also helped older ones bring in new funding and graduate students. Professor Sandor Vajda (BME, SE) and Paschalidis have been working together since before the establishment of the divisions, but have benefited from the collaborative space the divisions have created.
Their decades-long project involves building an automated server to predict how one protein might bind, or dock, to another one. Experimentally, this is an extremely difficult problem to solve in a biological lab, but by using computational methods, they have developed methodologies for predicting protein structures.
Vajda says that although this work has direct implications in the biomedical space, the work requires a strong background in mathematics and computational methods—a skill set commonly found in systems engineering graduate students. Now under SE, Vajda and Paschalidis can bring these students in to work on computationally heavy projects, and they don’t need a background in chemistry or biology. Instead of approaching the problem from a life sciences standpoint, they can approach it from another angle.
That versatility is what makes these two areas of research so encompassing. Paschalidis, for example, collaborates on projects that involve many different areas, including protein docking, optimizing travel routes to mitigate traffic, and predicting and monitoring radiation-exposure levels in hospitals.
Both of these far-reaching disciplines, although very different, are heavily involved in research to help solve the climate change crisis. SE researchers attempt to optimize smart grids to cut electricity usage, and MSE researchers are developing new materials for more efficient renewable energy generation and storage.
“I think the great research universities like BU have a moral obligation to work on the great problems,” says Bishop. “We’re lucky enough to live very comfortable lives, and I think that comes with the responsibility to focus on some of the big, important problems, which fundamentally require interdisciplinary research.”